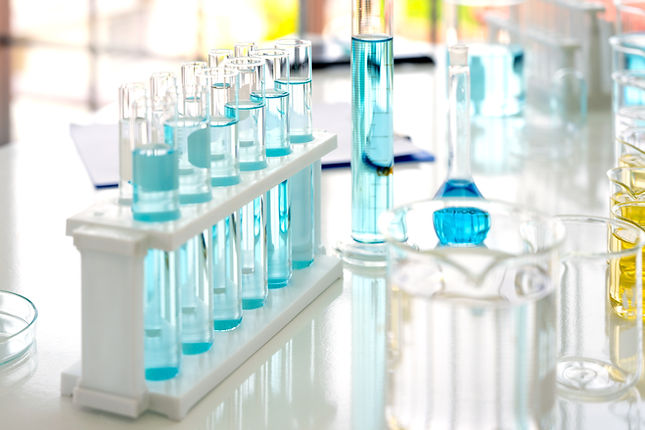
Research

The NSF Center for Aqueous Supramolecular Chemistry (CASC) is supported by the Centers for Chemical Innovation (CCI) Program of the Division of Chemistry. The ability of custom-designed molecules to 1) selectively recognize and bind to negatively charged molecules (anions), 2) transport these anions across membranes and/or 3) enable chemical transformations to new products is an all-but-unmet challenge. This Center will overcome these challenges by synthesizing novel molecules that target two anions of particular importance to society: bicarbonate and perfluorooctanoic acid (PFOA). Carbon dioxide from greenhouse gas emissions resides predominantly in surface ocean waters as bicarbonate. PFOA is well recognized for its persistence and toxic effects in groundwater. The selective capture, transport and transformation of these two anions will foster numerous technological payoffs. Activities within this Center include the training of students in the commercialization of technology and the creation of custom Individual Development Plans for all incoming scholars. CASC will establish a summer undergraduate program that trains students to continue projects at their home institution, and will engage the public in hands-on activities at museums and science centers.
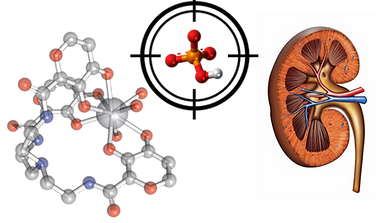
Hyperphosphatemia and kidney disease
Hyperphosphatemia, a condition that occurs when the phosphate concentration in serum exceeds 1.46 mM, is common among patients with advanced chronic and acute kidney disease (CKD) and kidney failure. Maintenance hemodialysis does not remove phosphate from blood; thus, almost all patients on maintenance hemodialysis have hyperphosphatemia. Current treatment relies primarily on dietary restrictions and the administration of oral phosphate binders with food or drink which are often insufficient to manage hyperphosphatemia for individuals on dialysis. This inability to manage the disorder increases morbidity, mostly due to cardiovascular events related to vascular and soft tissue calcification. Our group has designed and optimized a unique class of phosphate receptors that have high affinity for phosphate, exquisite selectivity over competing endogenous anions, and high stability. The overarching goal of this project is to develop, optimize, and translate to the clinic novel affinity columns functionalized with these receptors for normalizing the levels of inorganic phosphate from blood quickly, safely, and selectively.

Direct ocean capture of CO2
The role of carbon dioxide as a predominant greenhouse gas (GHG) in driving climate change is now beyond dispute. Because global advances in reducing net emissions of CO2 are proving insufficient, implementation of negative emission technologies is becoming imperative to counterbalance past and current emissions. Technologies currently employed for CO2 capture focus on gaseous CO2, and, due to its low concentration in the atmosphere, require strong binding. As a result, the costs of existing technologies to capture and recover CO2 are typically very high, hindering their large-scale adoption. Because oceans contain ca. 45 times more CO2 than the atmosphere, direct ocean capture (DOC) and other oceanic approaches are recognized as one of the key pillars for CDR. Given the alkalinity of the oceans (pH ≈ 8), carbon dioxide primarily exists in surface ocean water as bicarbonate (HCO3–) at relatively high concentrations (2.1 mM) but in the presence of many other anions, some of which are at considerable excess, notably chloride (560 mM) and sulfate (29 mM). Any solution for DOC requires high selectivity, and hence, it should be approached through supramolecular chemistry of the bicarbonate anion in water. Unfortunately, one consequence of the lack of attention to DOC is that this fundamental chemistry remains underexplored. Key questions remain unanswered, such as how does one capture, transport, release, and transform bicarbonate anion salts and how does one do so selectively in the presence of other competing species, such as chloride and sulfate? Addressing these questions could provide new energy-efficient technology for DOC.
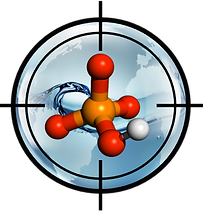
Switchable receptors for phosphate
The over-supply of phosphorus (P) primarily from wastewater discharge and agricultural runoff can lead to eutrophication in many inland and coastal waters, causing substantial detrimental environmental impact, including harmful algal blooms, fish-kills, and the formation of hypoxic “dead zones”. Over 65% of US estuaries and coastal waters now exhibit moderate to severe eutrophication, with significant ecological, industrial, and economic consequences. Removal of P from wastewater and agricultural runoff is key to mitigating eutrophication. Our overarching goal is to close the P cycle by sequestering phosphate (Pi) from polluted wastewater and waterways and recovering it as slow-release fertilizers. The ability to remove phosphate from unwanted locations and to recover it as a valuable resource for agriculture is key to the long-term sustainable use of two critical resources: water and phosphate. With this in mind, we previously designed and developed efficient inorganic receptors with high affinity for inorganic Pi that capture the anion directly in water at neutral pH, at the ppb level, and with very high selectively over competing anions such as chloride, sulfate and bicarbonate. We also developed a set of guiding principles that enable us to control and turn on and off at will the affinity of our receptor for Pi. We are now using these guiding principles to design new materials and polymers to sustainably capture and release phosphate from wastewater upon addition of an external physical or chemical stimulus.
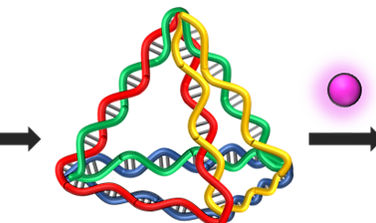
DNA-templated supramolecular synthesis of polymers
We are developing a new class of three-dimensional precision polymers. The unique wireframe topology of these hollow polymers open new possibilities in a number of applications including as solubilizing agents, as molecular and imaging probes, and as encapsulating and delivery agents. A unique aspect of the wireframe polymers is their templated synthesis. The approach employs predictive supramolecular strategies to template the position of each monomer on a DNA nanostructure. Subsequent coupling of the monomers yields the final desired macromolecule in one pot. The modularity of the templated approach will facilitate tuning of the polymer’s size, topology, and functional groups to match the needs of the intended applications.